Molecule of the Month: Designer Insulins
Engineered insulins have been developed to improve treatment of diabetes
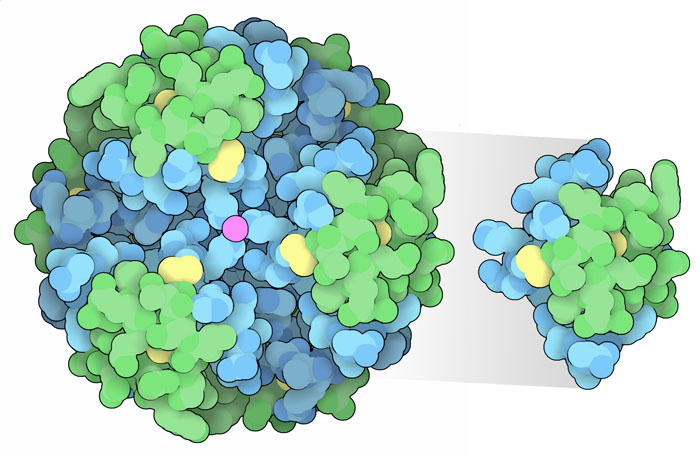
Tuning the Treatment
Long-acting Insulin
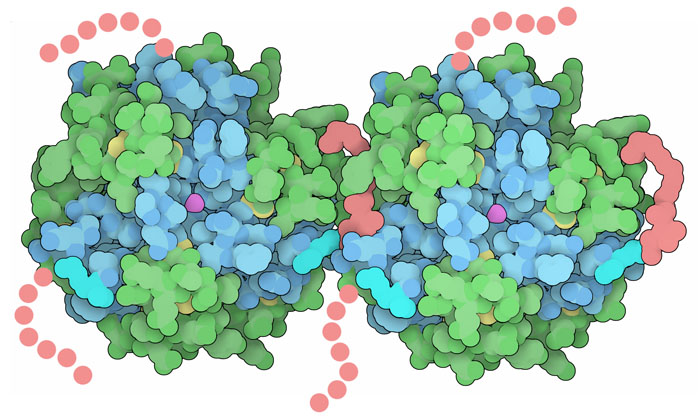
Tailoring Interactions
Exploring the Structure
Fast-acting Insulin (PDB entries 1tyl, 1lph, 4gbc)
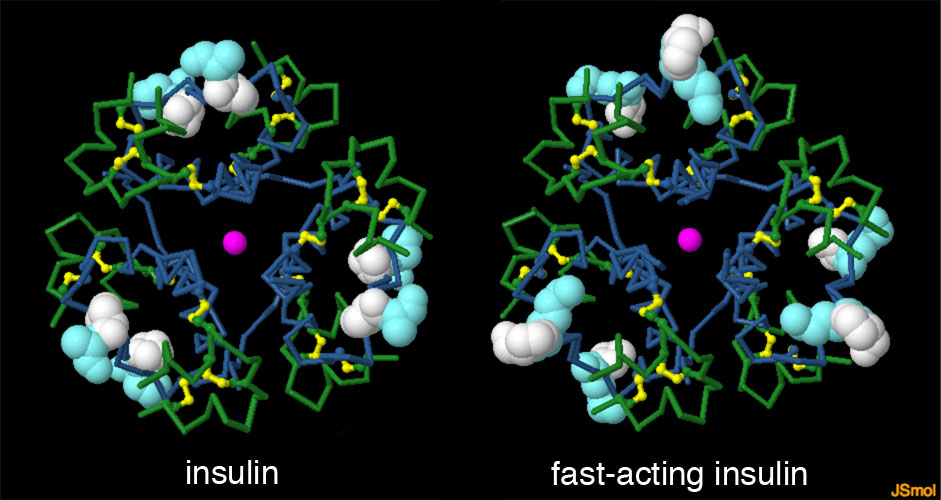
Long-acting insulins are combined with fast-acting insulins that are used immediately after meals. The molecular trick to creating a fast-acting insulin is to destabilize the insulin hexamer, so it falls apart rapidly when it enters the bloodstream. The first fast-acting design, lispro (Humalog ®), involved the tiniest of changes in the molecule, reversing the order of two residues near the C-terminus of the B-chain. This change weakens the interaction between insulin monomers, making the mutant hexamer 200 times less stable than the native form (PDB entry 1lph). Further study of the insulin structure showed that addition of a charged amino acid in the same location achieves the same outcome, yielding the fast-acting insulin aspart (Novolog ®) (PDB entry 4gbc). To explore these structures in more detail, click on the image for an interactive JSmol.
Topics for Further Discussion
- You can build a paper model of insulin—the template and an activity page are available on PDB-101.
- Surprisingly, small preservative molecules like phenol and cresol play an important role in insulin therapy, stabilizing the hexamer until it reaches the bloodstream. Many of the structures of insulin in the PDB archive include several of these molecules, stabilizing the interactions between the chains in the hexamer.
- Researchers are also looking for ways to design ultra-stable insulin molecules that have only a single chain. Look, for example, at PDB entry 2jzq.
Related PDB-101 Resources
- Browse Diabetes
- Browse Cellular Signaling
References
- J. Pettus, T. S. Cavaiola, W. V. Tamborlane & S. Edelman (2015) The past, present, and future of basal insulins. Diabetes/Metabolism Research and Reviews, DOI 10.1002/dmrr.2763.
- R. Hilgenfeld, G. Seipke, H. Berchtold & D. R. Owens (2014) The evolution of insulin glargine and its continuing contribution to diabetes care. Drugs 74, 911-927.
- 4gbc: L. C. Palmieri, M. P. Favero-Retto, D. Lourenco & L. M. T. R. Lima (2013) A T3R3 hexamer of the human insulin variant B28Asp. Biophysical Chemistry 173, 1-7.
- 4ajx: D. B. Steensgaard, G. Schluckebier, H. M. Strauss, M. Norrman, J. K. Thomsen, A. V. Friderichsen, S. Havenlund & I. Jonassen (2012) Ligand-controlled assembly of hexamers, dihexamers, and linear multihexamer structures by the engineered acylated insulin degludec. Biochemistry 52, 295-309.
- 1lph: E. Ciszak, J. M. Beals, B. H. Frank, J. C. Baker, N. D. Carter & G. D. Smith (1995) Role of C-terminal B-chain residues in insulin assembly: the structure of hexameric LysB28ProB29-human insulin. Structure 3, 615-622.
- 1trz: Ciszak, E. & G. D. Smith (1994) Crystallographic evidence for dual coordination around zinc in the T3R3 human insulin hexamer. Biochemistry 33, 1512-1517.
- 1tyl: G. D. Smith & E. Ciszak (1994) The structure of a complex of hexameric insulin and 4’-hydroxyacetanilide. Proceedings of the National Academy of Sciences USA 91, 8851-8855.
February 2016, David S. Goodsell
http://doi.org/10.2210/rcsb_pdb/mom_2016_2