Molecule of the Month: DNA-Sequencing Nanopores
Designer nanopores work with enzymes to offer pocket-sized DNA sequencing
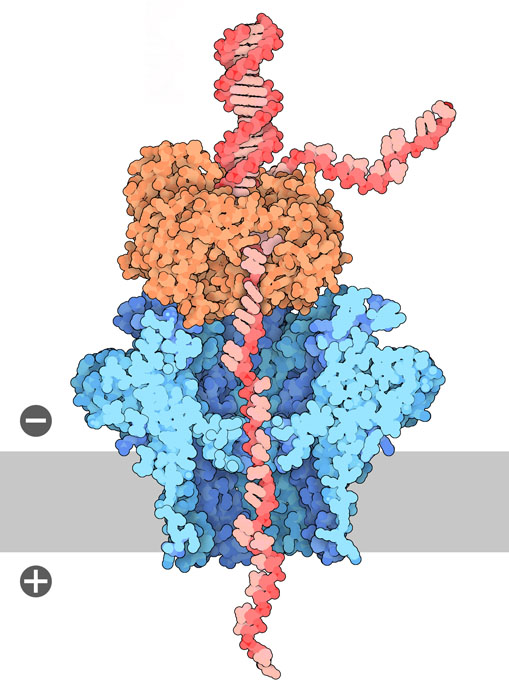
Pocket-sized Sequencers
Nanopores in Action
Regulating DNA Threading
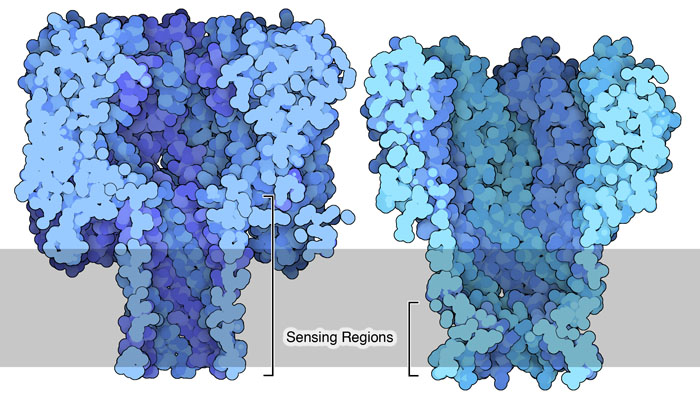
Nature’s Pores
Exploring the Structure
Dual constriction nanopores in action
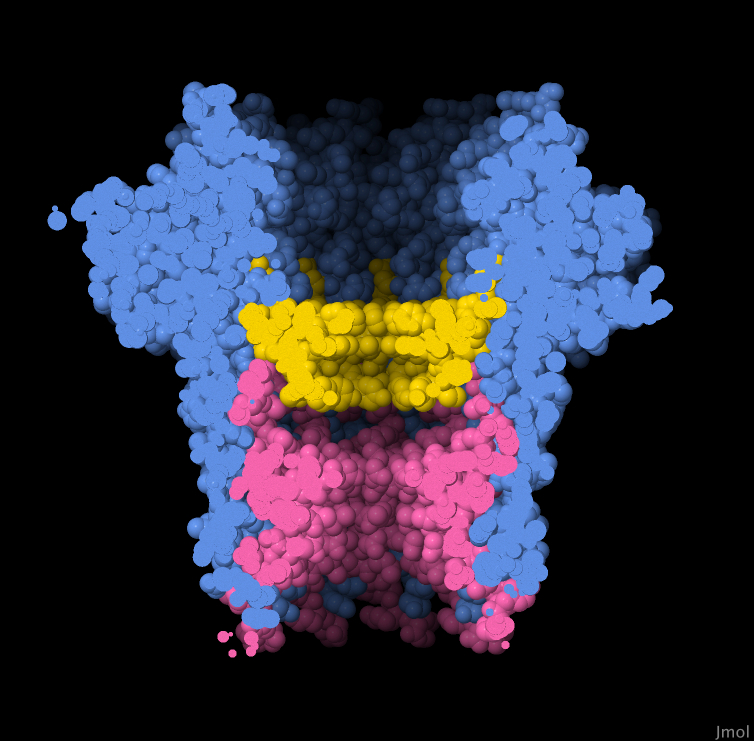
Scientists have engineered nanopores with greater pore stability and tuned for optimal function. For example, dual constriction nanopores add an accessory protein to CsgG to introduce a second constriction for detecting DNA. The CsgG-CsgF complex shown here (PDB ID 6si7) highlights the CsgG molecule in blue with its pore-forming region in yellow, and the CsgF molecule in pink. CsgG forms the initial constriction point for DNA and CsgF adds a second constriction. Integrating the signal from two constriction sites improves base-reading accuracy, particularly in DNA stretches with multiple consecutive copies of the same base. Click on the image to explore this structure in an interactive JSmol.
Topics for Further Discussion
- If you want to explore the structure of DNA bases, PDB ID 2rpd includes a single DNA strand with one of each of the four types of bases.
- Aerolysin is another commonly used nanopore sensor borrowed from a bacterium. You can see the wild-type pore in the PDB ID 5jzt.
Related PDB-101 Resources
- Browse Biotechnology
- Browse Nanotechnology
References
- 6si7: Van der Verren, S.E., Van Gerven, N., Jonckheere, W., Hambley, R., Singh, P., Kilgour, J., Jordan, M., Wallace, E.J., Jayasinghe, L., Remaut, H. (2020) A dual-constriction biological nanopore resolves homonucleotide sequences with high fidelity. Nat Biotechnol 38, 1415-1420.
- Carter, J.M., Hussain, S. (2017) Robust long-read native DNA sequencing using the ONT CsgG Nanopore system. Wellcome Open Res, 2, 23.
- Castro-Wallace, S.L., Chiu, C.Y., John, K.K., Stahl, S.E., Rubins, K.H., McIntyre, A.B.R., Dworkin, J.P., Lupisella, M.L., Smith, D.J., Botkin, D.J., Stephenson, T.A., Juul, S., Turner, D.J., Izquierdo, F., Federman, S., Stryke, D., Somasekar, S., Alexander, N., Yu, G., Mason, C.E., Burton, A.S. (2017) Nanopore DNA sequencing and genome assembly on the International Space Station. Sci Rep 7, 18022.
- Johnson, S.S., Zaikova, E., Goerlitz, D.S., Bai, Y., Tighe, S.W. (2017) Real-time DNA sequencing in the Antarctic dry valleys using the Oxford Nanopore sequencer. J Biomol Tech 28, 2-7.
- Deamer, D., Akeson, M., Branton, D. (2016) Three decades of nanopore sequencing. Nat Biotechnol 34, 518-524.
- 4uv3: Goyal, P., Krasteva, P.V., Van Gerven, N., Gubellini, F., Van den Broeck, I., Troupiotis-Tsailaki, A., Jonckheere, W., Pehau-Arnaudet, G., Pinkner, J.S., Chapman, M.R., Hultgren, S.J., Howorka, S., Fronzes, R., Remaut, H. (2014) Structural and mechanistic insights into the bacterial amyloid secretion channel CsgG. Nature 516, 250-253.
- Lieberman, K.R., Cherf, G.M., Doody, M.J., Olasagasti, F., Kolodji, Y., Akeson, M. (2010) Processive replication of single DNA molecules in a nanopore catalyzed by phi29 DNA polymerase. J Am Chem Soc 132, 17961–17972.
- 1uun: Faller, M., Niederweis, M., Schulz, G.E. (2004) The structure of mycobacterial outer-membrane channel. Science 303, 1189-1192.
- 1xhz: Kamtekar, S., Berman, A.J., Wang, J., Lazaro, J.M., de Vega, M., Blanco, L., Salas, M., Steitz, T.A. (2004) Insights into strand displacement and processivity from the crystal structure of the protein-primed DNA polymerase of bacteriophage phi29. Mol Cell 16, 609-618.
- 7ahl: Song, L., Hobaugh, M.R., Shustak, C., Cheley, S., Bayley, H., Gouaux, J.E. (1996) Structure of staphylococcal alpha-hemolysin, a heptameric transmembrane pore. Science 274, 1859-1866.
September 2021, Jennifer Jiang, Katherine H. Park, Kiranmayi Vemuri, David Goodsell
http://doi.org/10.2210/rcsb_pdb/mom_2021_9