2022 Video Challenge for High School StudentsMolecular Mechanisms of Cancer |
2022 Video Challenge
Winners | Entries | Judges | Overview | Learning Resources
Note: Videos are intended for communication and not rigorous scientific review.
Congratulations to the 2022 Winners:
Summary of the 2022 Video Challenge
Cancer is caused by cells that have lost their normal controls on growth, and in the worst cases, have developed ways to move throughout the body. Since all of our cells must work together to create a healthy body, cell growth and the cell division cycle are regulated by complex molecular systems. The components of these systems – the molecules and proteins – are arranged into pathways where sequential positive and negative feedback events cause the cell cycle to proceed or stop at the appropriate times. In cancer cells, this feedback system is disrupted and they grow uncontrollably, forming a tumor.
These disruptions are often caused by mutations in genes that encode signaling proteins. These mutated genes then produce mutated proteins that are structurally altered and function improperly within the signaling pathway. Many of the genes that carry these carcinogenic mutations can be divided into 2 groups: tumor suppressor genes and proto-oncogenes.
Tumor suppressor genes encode proteins that regulate and control growth. These genes watch for problems and shut down growth and initiate repairs when they find them. For example, some tumor suppressors respond to DNA damage. They act as transcription factors stimulating production of proteins that help in DNA repair as well proteins that arrest division cycle until DNA is repaired or induce cell death if repair is not possible. If there is a mutation at a critical location in a tumor suppressor gene, the expressed protein has an altered 3D structure which renders it unable to react to issues with DNA. As a consequence, the cells with DNA damage can proliferate.
Proto-oncogenes, on the other hand, help control the processes that are essential for cell division. These proteins are integral parts of specific pathways and rely on positive signals from other proteins and molecules to become activated and to pass the signal forward. A mutated proto-oncogene is referred to as oncogene. Oncogenes produce proteins with structural changes that allow them to be active independent from feedback from other proteins, so they continually tell the cell that it's okay to grow and divide.
In this video challenge we would like you to explore two specific molecular feedback systems that have an important role in the cell division cycle: the p53/p21 pathway and the EGFR/Ras pathway. Mutations in the TP53 gene (encoding the p53 tumor suppressor protein) are identified in 50% of all cancers, while mutations in the RAS gene (encoding the Ras protein) are identified in 30% of all cancers.
Your task is to create a 2 minute-long video that tells a coherent story explaining the science concepts from one of the topics below and touches on the public health aspects of cancer such as screening, prevention, and awareness.
TOPIC 1:
Failure of cell-cycle arrest via p21 protein due to mutations in the TP53 tumor suppressor gene.
Your video should communicate the following scientific concepts:
- What is the function of the p53 protein? How can it modulate the cell cycle if necessary?
- How can a mutation in the TP53 gene affect the function of the p53 protein?
- How does mutation of the p53 protein impact the function of p21, leading to cancer?
TOPIC 2:
Continuous cell proliferation due to mutations in the RAS oncogene in the EGFR/Ras pathway
Your video should communicate the following scientific concepts:
- What is the function of the Ras protein in the cell growth pathway?
- How can a mutation in the RAS gene affect the function of Ras protein and cause disruption in the signaling pathway, leading to cancer?
2022 Video video-challenge Dates
Submission Opened | January 18, 2022 |
Submission Closed | April 25, 2022 at 11:59 pm PST |
Judging and Voting | May 3 - May 9, 2022 |
Results | Award winners were announced at rcsb.org and pdb101.rcsb.org on May 17, 2022. |
2022 Learning Resources
Topic 1 overview:
Failure of cell-cycle arrest via p21 protein due to mutations in the TP53 tumor suppressor gene
Notice: This topic overview specifically highlights the proteins and their functions that are relevant to the p53/p21 pathway. Most of the proteins described here are essential components in other pathways.
Understanding the cell division cycle
The cell division cycle is a series of sequential events that lead to a cell splitting into 2 daughter cells. This process follows distinct phases: G1, S, G2, and M. In the G1 phase, the cell is increasing its supply of proteins relevant for DNA replication and copying its organelles. In the S phase, the cell replicates its DNA. In the G2 phase, the cell continues to grow, preparing itself for mitosis by reorganizing its microtubules to complete the division in the M phase (see Figure 1, outer ring).
Figure 1. Cell division cycle with phase-specific cyclins and CDKs and their main functions highlighted.
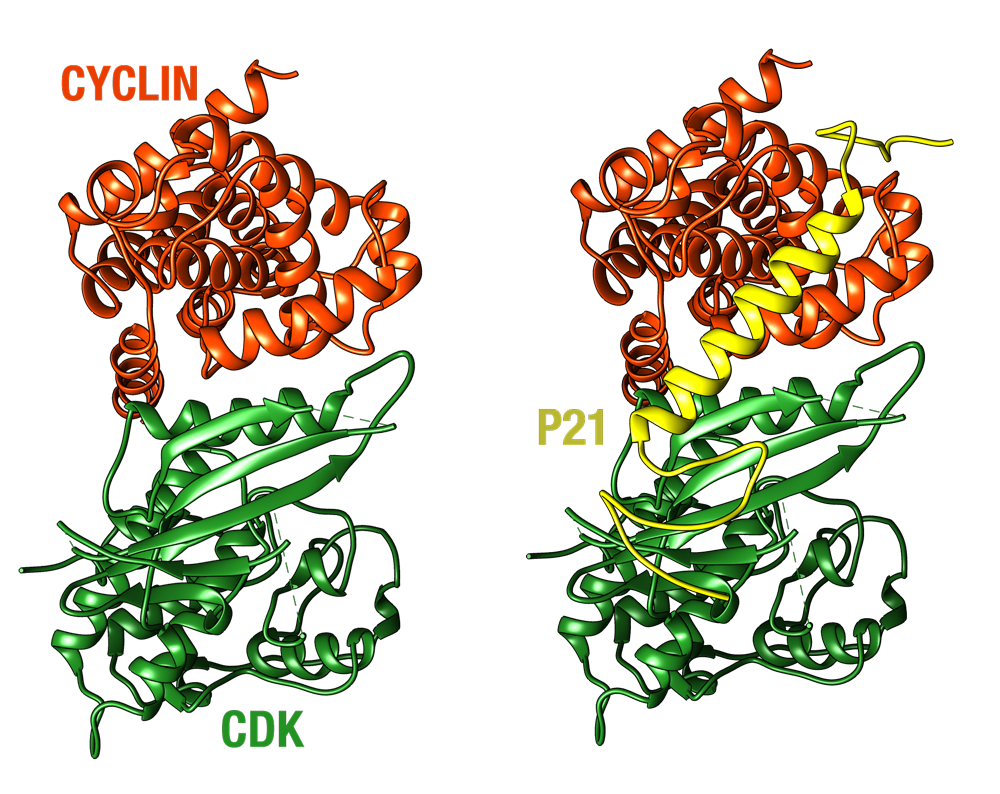
Figure 2. Active CDK (green)/cyclin (orange) complex is shown on the left. The same complex is shown on the right inactivated by the p21 protein (yellow). PDB structure 6p8h.
The cell cycle progression is mediated through various cyclins and cyclin-dependent kinases (CDKs). Kinases are a large family of proteins that can phosphorylate other proteins. Phosphorylation is a process of adding phosphoryl groups to certain amino acids (see Figure 4A). This process can activate or deactivate the protein. CDKs have to create a complex with another protein – cyclin – to be able to phosphorylate other proteins. The levels of various phase-specific CDKs are relatively stable throughout the cell cycle while the concentrations of phase-specific cyclins rise and fall. Figure 1 shows the phase-specific cyclins and CDKs and their key functions in the progression of the cell cycle.
The protein p53 is activated when DNA damage is detected. p53 triggers expression of the p21 protein. p21 inhibits CDK/cyclin complexes (see Figure 2) pausing the cell cycle progression and giving the cell time to repair its DNA or start pathways leading to its death.
Learn More:
Molecule of the Month articles:
Cyclin and Cyclin-dependent Kinase
Other Resources:
L. Ding et al. (2020) The Roles of Cyclin-Dependent Kinases in Cell-Cycle Progression and Therapeutic Strategies in Human Breast Cancer. Int J Mol Sci. 21(6): 1960.
https://www.ncbi.nlm.nih.gov/pmc/articles/PMC7139603/
L. Wang et al. (2020) Function of p21 and its therapeutic effects in esophageal cancer (Review). Oncology Letters 21:2.
https://www.spandidos-publications.com/10.3892/ol.2020.12397
The p53 Tumor Suppressor
p53 is a transcription factor that responds to cell stress events that can result in DNA damage. p53 initiates and stimulates the production of proteins that assist in DNA repair, arrest the cell-division cycle, or initiate apoptosis. p53 is composed of four identical chains. At the center of p53 is a tetramerization domain that ties the four chains together. Each of the chains features a DNA-binding domain and a transactivation domain (see Figure 3 A).
The protein receives positive and negative stimulation through post-translational modifications (e.g. phosphorylation, acetylation, methylation and ubiquitination).
Negative control of p53
When there are no signals about DNA damage, MDM2 is bound to the transactivation domain of p53, preventing the protein from becoming activated (see Figure 3B).
Figure 3. A) the structure of p53 with the main domains highlighted. The illustration was created by overlaying PDB structures (1ycq-transactivation domain, 1sae-tetramerization domain, 3q01-DNA-binding domain) on the AlphaFold21computational model. B) p53 is expressed at steady levels. If DNA damage is not detected, MDM2 (PDB structure 1ycq) binds to the transactivation domain, marking the protein for destruction.
Positive stimulation of p53 resulting in production of p21
Cellular stresses such as UV light, ionizing radiation, and other factors can cause breaks in DNA strands. The DNA damage activates complex molecular pathways. One of the proteins activated through such pathways is the CHK2 kinase. The protein can phosphorylate the p53 transactivation domain at the Serine 20 site (see Figure 4A). This activates p53 causing it to bind to DNA. p53 typically binds to special sequences in the target genes called p53 response elements.
Figure 4. A) DNA damage activates complex molecular pathways. One component of them is CHK2 kinase (green, PDB structure 2cn5). The kinase phosphorylates p53 at the Serine 20 site. B) Phosphorylation activates p53 causing it to bind to a special segment of DNA called the p53 response element (PDB structure 3ts8).
One of the target genes for p53 is the CDKN1A gene encoding the p21 protein. After binding to the DNA, p53 engages the RNA Polymerase II (see Figure 5). The polymerase then transcribes the p21 gene into mRNA, starting the process leading to production of the p21 inhibitor. p21 then inhibits the CDK/cyclin complexes (see Figure 2) thus halting the progression of the cell cycle in the face of DNA damage present.
Figure 5. p53 engaging RNA Polymerase II (illustration created based on PDB structures 3ts8, 5iy6, and 6xre).
If a mutation is present at the DNA binding site, p53 can’t bind to DNA and initiate the p21 production. As a result, the cell will divide with errors in the DNA code.
Learn More:
Molecule of the Month articles:
Other Resources:
SnapShot: p53 Posttranslational Modifications
P53 Tumor Suppressor by whei.tv (Video)
The Role of p53 in Cancer (Video)
Table 1: Examples of relevant PDB structures for Topic 1
PDB Structure ID |
Structure Description |
Visualization Resources |
CyclinA-CDK2 complex |
Chimera session (preview below)b The Chimera session preview for the PDB structure 1w98 shows the CDK2 (green, chain A)/cyclin E (orange, chain B) complex. More examples of 3D structures of phase-specific cyclin and CDKs from the PDB can be found in Figure 1. |
|
Crystal structure of CDK4 in complex with Cyclin D and p21 |
Chimera session (preview below)b The Chimera session preview for the PDB structure 6p8h shows the CDK (green, chain B)/cyclin (orange, chain A) complex inactivated by the p21 protein (yellow, chain C). |
|
Crystal structure of a multidomain human p53 tetramer bound to the natural CDKN1A(p21) p53-response element |
Chimera session (preview below)b The Chimera session preview for the PDB structure 3ts8 shows the DNA-binding domains of p53 in cyan (chains A, B, C, and D) and the CDKN1A(p21) p53-response element in orange. |
|
Structure of the p53/RNA polymerase II assembly |
Chimera session (preview below)b The Chimera session preview for the PDB structure 6xre shows the RNA Polymerase II in dark red and one p53 DNA binding domain in cyan with the transactivation domain highlighted in green (chain M). |
Topic 2 overview:
Continuous cell proliferation due to mutations in the RAS oncogene in the EGFR/Ras pathway
Epidermal growth factor (EGF) and its receptor
Growth factors are required to initiate the G1 phase of the cell cycle. One such ligand is epidermal growth factor (EGF). The small peptide can bind to the extracellular portion of the EGF receptor (EGFR). Inactive EGFRs exist as monomers in cellular membranes. The receptor consists of an extracellular module, a membrane helix, and an intracellular kinase domain that features a long C-terminal tail studded with tyrosine residues (see Figure 6A). EGF binding induces the dimerization of the EGFRs. This process activates the kinase domains. The kinases now can bind ATP and tyrosine residues from the C-terminal tails and phosphorylate the tyrosine residues (see Figure 6B).
Learn more:
Molecule of the Month article:
Other Resources:
A. Arkhipov et al. (2013) Architecture and Membrane Interactions of the EGF Receptor. Cell 152(3): 557-569.
https://www.cell.com/fulltext/S0092-8674(12)01552-8
Figure 6. A) The inactive EGFR receptors are monomers (shown in cyan and blue). The receptor comprises an extracellular module (PDB structure 1nql) , a membrane helix (shown from PDB structure 5lv6), and an intracellular kinase domain (3gt8). The kinase features a long C-terminal tail with multiple tyrosine residues (shown on the model in flat render and lighter green). In the monomer form, the kinase is inactive. B) Upon EGF binding, the receptors dimerize. The model shown consists of PDB structures 3njp-extracellular modules, 5lv6-membrane helices, and 2gs6-activated kinases. The kinases bind ATP (highlighted in magenta) along with tyrosine residues from the tail and phosphorylate them. Added phosphoryl groups are shown in orange and red.
Activation and inactivation of ras protein
Ras is a small, globular protein that can be switched on or off by binding GTP and GDP respectively.
The activation of ras is dependent on the phosphorylation of the C-terminal tails of the kinase domain in EGFR. The adaptor protein GRB2 (Figure 7A) binds to the tail and recruits SOS (Figure 7B). SOS binds the inactive ras protein with GDP in its active site (Figure 7C). Upon binding, SOS opens the binding site of ras, allowing the exchange of GDP for GTP (Figure 7D). This exchange activates ras, allowing it to activate the raf kinase and to progress the pathway that ultimately leads to expression of various proteins essential in the cell division cycle, including cyclin D (Figure 7E).
Ras can break down the GTP on its own, however the process is slow. It is accelerated by ras-specific GTPase-activating proteins (rasGAP, Figure 7E). These enzymes hydrolyze the GTP to GDP. For details on the hydrolyzation reaction, see the Exploring the Structure section of the Molecule of the Month article on Ras Protein.
Mutation in the ras protein disallow the completion of the hydrolyzation reaction causing ras to stay in the ‘on’ state and constantly promote gene expression and cell growth.
Figure 7. A) The phosphorylated tail of the kinase binds adaptor protein GRB2 (shown from PDB structures 1gq1, 3gbq, and 1gfc). B) The N-terminal domain of GRB2 (1gq1) binds SOS (shown combined from PDB structure 6cuo and Alphafold1 model–in flat render). C), D) SOS then binds ras protein (6cuo) which allows the GDP – GTP exchange. E) Activated ras proceeds to activate raf kinase, progressing the pathway leading to expression of transcription activators. F) Ras is inactivated by rasGAP (PDB structure 1wq1).
Table 2: Examples of relevant PDB structures for Topic 2
PDB Structure ID |
Structure Description |
Visualization Resources |
Inactive EGF Receptor Model |
1nql, 5lv6, and 3gt8 3D views from Mol*a Chimera session (preview below)b This Chimera session contains the EGFR model constructed from three PDB structures: 1nql (extracellular module), 5lv6 (membrane helix), and 3gt8 (inactive kinase). To access and select the individual structures, use the Model Panel available from Favorites > Model Panel. From the panel you can show and hide the surfaces and select individual structures (use the 'select' button on the right). The cell membrane is indicated in the preview image for clarity. |
|
Activated EGF Receptor Model |
3njp, 5lv6, and 2gs6 3D views from Mol*a Chimera session (preview below)b This Chimera session contains the activated EGF receptor model constructed from three PDB structures. The extracellular dimerized module is shown from PDB structure 3njp, chains A and B. Each of them has an EGF peptide bound, shown in red (chains C and D). Membrane helices are shown from structure 5lv6, chains A and B. The activated kinase is shown from structure 2gs6 (biological unit). Each of the kinases has an ATP-like ligand bound in the active site (ligand ID 112, pink). |
|
Ras with GTP and GDP |
5p21 and 4q21 3D views from Mol*a Chimera session (preview below)b This Chimera session features two ras proteins. Structure 4q21 on the left shows the protein with GDP bound (ligand GDP). Structure 5p21 on the right shows ras with an ATP-like ligand bound (ligand GNP). |
|
RasGAP Complex |
Chimera session (preview below)b PDB structure 1wq1 shows the rasGAP complex. In this Chimera session, ras is colored orange (chain G) and has GTP in the active site. The GAP protein is shown in pink (chain G). |
References
- J. Jumper et al. Highly accurate protein structure prediction with AlphaFold. Nature (2021).
Visualization Resources References:
- You can download and open these sessions using UCSF Chimera. Use the UCSF Chimera tutorial to learn how to edit the sessions, create animations, or save pictures.
- Mol* is a web-based molecular viewer. Use the video tutorial Exploring PDB Structures in 3D with Mol*: Introductory Guide to get started with this molecular viewer. Access the full documentation learn about more advanced features. Mol* is accessible from each structure summary page, from the tab “3D View”.