Molecule of the Month: Histone Deacetylases
Histone deacetylases regulate access to genetic information by modifying histones
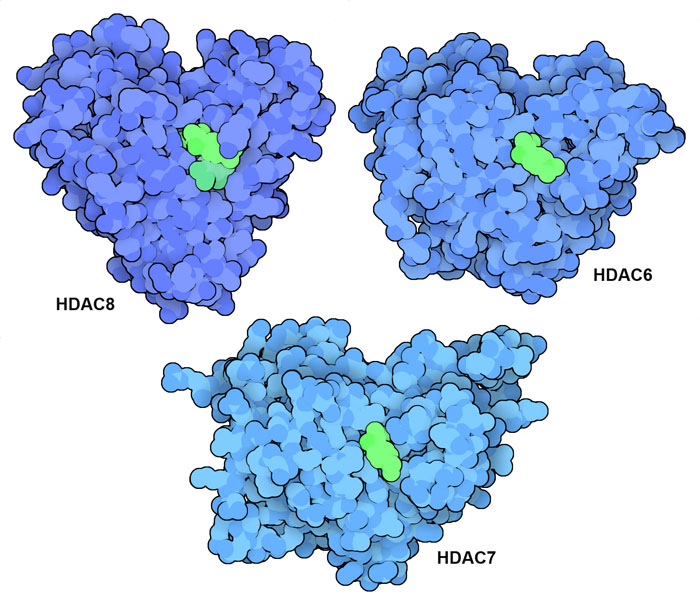
The Deacetylation Supervisor
Targeting HDAC
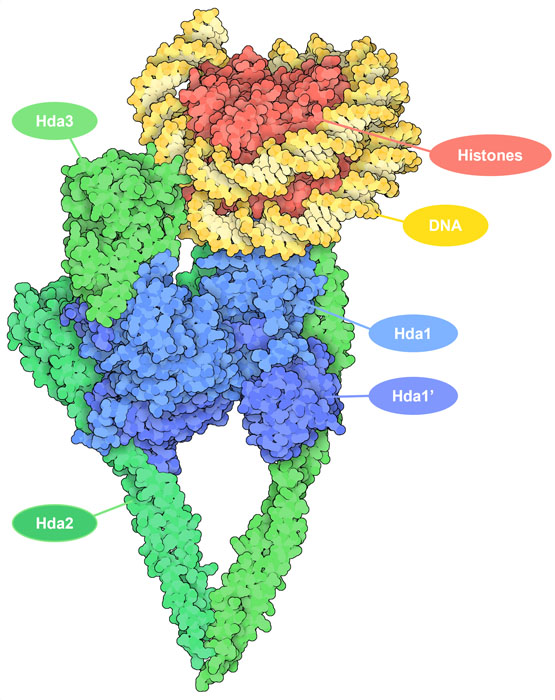
Working as a Team
Exploring the Structure
HDAC8 with Substrate and Inhibitors
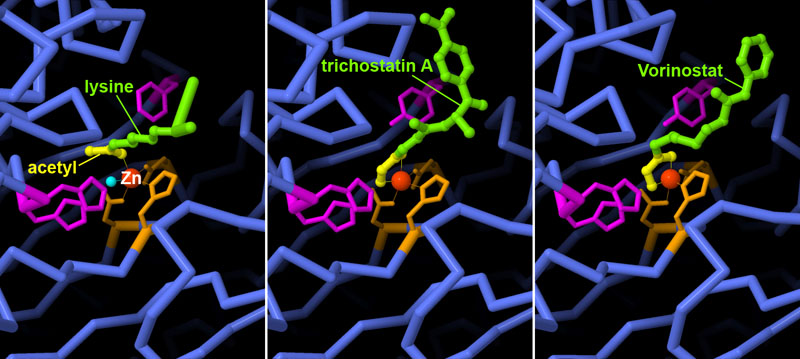
Various HDAC inhibitors are currently being used as anticancer drugs. They act by mimicking the natural target of HDACs: an acetylated lysine amino acid. PDB ID 2v5w shows how HDAC8 recognizes the lysine. A zinc ion coordinates the acetyl group (in yellow), positioning the molecule next to several amino acids (shown in magenta here) and a water molecule (turquoise) that help with the deacetylation reaction. The inhibitors have a chemical group that mimics both the acetyl group and the water, clamping the molecule tightly to the zinc ion, as seen in the structure of trichostatin A (PDB ID 1t64), a natural product isolated from Streptomyces hygroscopicus, and the US-FDA approved anticancer drug Vorinostat (PDB ID 1t69). To explore these structures in more detail, click on the image for an interactive JSmol.
Topics for Further Discussion
- You can use the structure comparison tool to explore the similarities and differences between different HDACs, such as HDAC6 (5edu), HDAC7 (3c10), and HDAC8 (1t64).
- The histone tails are very flexible, so coordinates for them are not usually included in the atomic coordinate files stored in the PDB. You can use the sequence view to determine what parts of the protein are represented in each PDB files, for example, the page for histone H3.2. Take a look at PDB ID 1kx5 for a particularly complete structure.
Related PDB-101 Resources
- Browse Cancer
- Browse Central Dogma
- Browse Drug Action
References
- 1t64, 1t69: Somoza, J. R., Skene, R. J., Katz, B. A., Mol, C., Ho, D. J., Jennings, A. J., Luong, C., Arvai, A., Buggy, J. J., Chi, E., Tang, J., Sang, B., Verner, E., Wynands, R., Leahy, E. M., Dougan, D. R., Snell, G., Navre, M., Knuth, M. W., Swanson, R. V., McRee, D. E., Tari, L. W. (2004) Structural snapshots of human HDAC8 provide insights into the Class I histone deacetylases. Structure 12: 1325–1334.
- 2v5w: Vannini, A., Volpari, C., Gallinari, P., Jones, P., Mattu, M., Carfi, A., Defrancesco, R., Steinkuhler, C., Di Marco, S. (2007) Substrate binding to histone deacetylases as revealed by crystal structure of Hdac8-substrate complex. EMBO Rep 8: 879-884.
- 3c10: Min, J., Schuetz, A., Loppnau, P., Weigelt, J., Sundstrom, M., Arrowsmith, C.H., Edwards, A.M., Bochkarev, A., Plotnikov, A.N., Structural Genomics Consortium (SGC) (2008) Crystal structure of catalytic domain of human histone deacetylase HDAC7 in complex with trichostatin A (TSA). J Biol Chem 283: 11355-11363.
- 5edu: Hai, Y., Christianson, D.W. (2016) Crystal structure of human histone deacetylase 6 catalytic domain 2 in complex with trichostatin A. Nat Chem Biol 12: 741-747.
- 6z6p: Lee, J.-H., Bollschweiler, D., Schafer, T., Huber, R. (2021) Structural basis for the regulation of nucleosome recognition and HDAC activity by histone deacetylase assemblies. Sci Adv 7: eabd4413.
- Delcuve, G. P., Khan, D. H., Davie, J. R. (2012). Roles of histone deacetylases in epigenetic regulation: emerging paradigms from studies with inhibitors. Clin Epigenetics 4:5.
- Kim, E., Bisson, W.H., Lohr, C.V., Williams, D.E., Ho, E., Dashwood, R.H., Rajendran, P. (2016) Histone and non-histone targets of dietary deacetylase inhibitors. Curr Top Med Chem 16, 714-731.
- Li, Y., Seto, E. (2016) HDACs and HDAC Inhibitors in cancer development and therapy. Cold Spring Harb Perspect Med 6: a026831.
- Hai, Y., Shinsky, S.A., Porter, N. J., Christianson, D.W. (2017) Histone deacetylase 10 structure and molecular function as a polyamine deacetylase. Nat Comm 8:15368.
- Milazzo, G., Mercatelli, D., Di Muzio, G., Triboli, L., De Rosa, P., Perini, G., Giorgi, F. M. (2020) Histone deacetylases (HDACs): Evolution, specificity, role in transcriptional complexes, and pharmacological actionability. Genes (Basel) 11: 556.
- Park, S., Kim, J. (2020) A short guide to histone deacetylases including recent progress on class II enzymes. Exp Mol Med 52: 204–212.
September 2023, Jessica Damanski, Paloma Munguía Salazar, Mihika Shah, Rajiv Snape, David S. Goodsell, Stephen K. Burley
http://doi.org/10.2210/rcsb_pdb/mom_2023_9